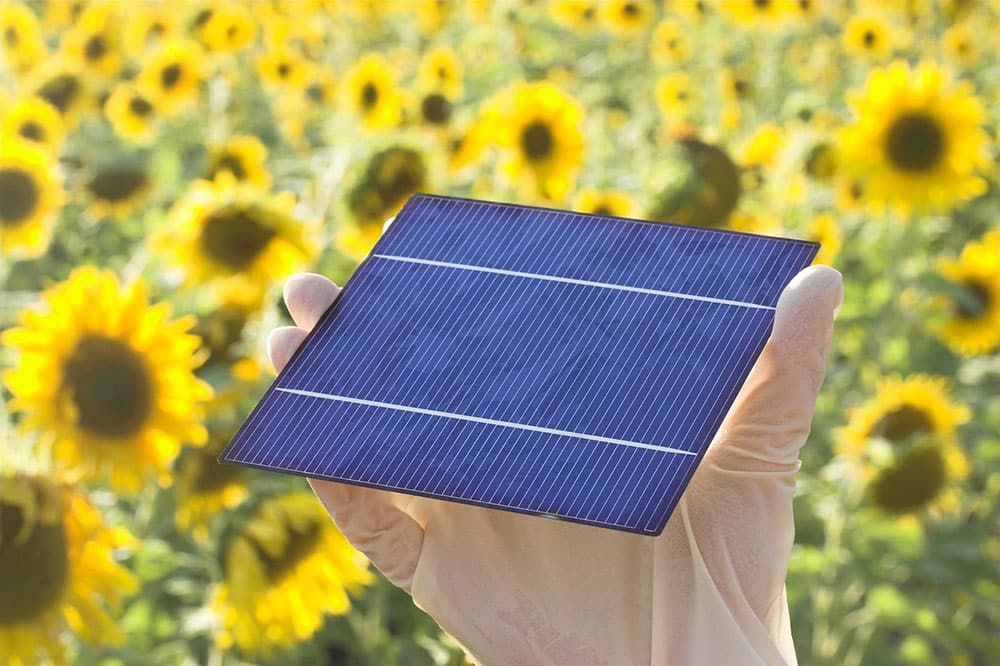
Basic Principles of Photovoltaics
Solar energy isn’t new. People have tried to harness it for ages. Nowadays, with the push to go green and be eco-friendly, solar energy is big news. But how do we turn the sun’s rays into usable power? That’s where photovoltaic (PV) technology comes in. PV tech takes the sun’s energy and changes it into electricity.
So, what’s the secret behind photovoltaics? How does it really work? Let’s break it down.
At its core, photovoltaic is about turning light into electricity. It’s a bit of science magic. When light hits certain materials, it can create an electric current.
Let’s talk photons. They’re tiny packets of light energy. When the sun’s rays hit the solar cell, they’re packed with these photons. Now, every material reacts to light differently. The special thing about PV materials is that they can absorb these photons.
When these photons get absorbed by the solar cell, they transfer their energy. This energy then pushes electrons out of their usual spots. Now, these electrons don’t just wander off. They start moving in a specific direction. This movement creates an electrical current.
Now, think about a solar panel you might have seen on a roof. It’s not just one cell. It’s many. When photons hit all these cells, a lot of electrons start moving. All these moving electrons create a flow. This flow is what we call electricity.
But there’s a catch. The electricity from solar cells is direct current (DC). Most of our homes and devices need alternating current (AC). So, there’s an extra step. But we’ll dive deeper into that later.
For now, just remember this: Photovoltaics is all about catching and using the sun’s energy. Photons hit the cell. Electrons move. Electricity gets made. And just like that, the sun’s rays power our lives.
Components and Structure of a Solar Cell
Breaking Down the Layers of a Solar Cell
Let’s get to the meat of it. A solar cell isn’t just one simple piece. It’s made up of layers, each with its own job. Picture a sandwich. Every ingredient plays a part. It’s the same with solar cells.
- Top Layer: This is the front of the solar cell, the part that faces the sun. It’s usually made of a clear material that lets sunlight in but protects the inside.
- Antireflective Coating: Sunlight can bounce off things. This layer stops that from happening too much. Instead of bouncing off, sunlight goes into the cell. This means more energy gets used.
- Emitter: Right under the top is the emitter. It’s made of a type of material that grabs the sun’s energy. When the energy hits it, electrons get excited.
- Base: The base is the thickest layer. It supports the whole cell and has the job of collecting the electrons that get excited by the sun’s energy.
- Back Layer: This is the solar cell’s rear side. It might have metal on it. This metal helps move the electricity out of the cell and into where it needs to go.
The Role of Semiconductors and How They Facilitate Electron Flow
Okay, so remember when we talked about the sun’s energy making electrons move? That’s where semiconductors come in. They’re the heart of the solar cell. Let’s get into why they’re so important.
Semiconductors are special materials. They’re in between conductors (like metal) and insulators (like rubber). Sometimes they let electricity flow. Sometimes they don’t. It all depends on the energy they get.
Imagine a busy street with lots of people. Now imagine a dance floor. On the street, people are just walking. But on the dance floor, with the right music (or energy), those people start moving and grooving. Semiconductors are like that dance floor. When the sun’s energy hits them, electrons start dancing.
Here’s how it works:
- The sun shines.
- Photons (from the sun) hit the semiconductor.
- Electrons in the semiconductor get energy from the photons.
- These electrons start to move. They want to get away from where they were.
- When many electrons move together, that’s a current.
- We capture this current, and we’ve got electricity.
Now, not all semiconductors are the same. Some are made to have extra electrons. Some are made to have fewer. When we put these two types together in a solar cell, it creates a special area called the “junction.” This junction is where the real magic happens. It’s where the sun’s energy makes the biggest difference, pushing electrons one way and creating an electric current.
Types of Solar Cells and Their Efficiency
Differentiating Between Monocrystalline, Polycrystalline, and Thin-Film Cells
Think of solar cells like types of bread. There’s more than one kind, and each has its own flavor and use. Similarly, solar cells come in different types: monocrystalline, polycrystalline, and thin-film. Let’s break them down.
- Monocrystalline: Fancy word, right? Think “mono” for “one.” These cells are made from a single, pure crystal structure. They’re usually black or deep blue and have rounded corners. They’re the “classic” solar cell you might picture.
- Polycrystalline: “Poly” means many. So, unlike monocrystalline, these cells are made from multiple crystal structures melted together. They’re usually blue and have a squared look. They’re like the younger sibling of the monocrystalline cells.
- Thin-Film: These are different. Instead of big panels like the other two, thin-film cells are, well, thin. Imagine a sheet of paper. They’re made by placing layers of photovoltaic material on a substrate. They can be super flexible and are often used on curved surfaces or even in foldable portable chargers.
How the Structure of Each Type Affects Its Efficiency
Each type of cell has its own “personality” when it comes to efficiency. It’s like how different cars have different fuel efficiencies.
- Monocrystalline Cells: The purebred of solar cells. Because they’re made from one crystal, they have fewer imperfections, which means electrons can move easily. This makes them the most efficient of the three, usually between 15%-20%. But with higher efficiency comes a higher price tag.
- Polycrystalline Cells: These guys are a bit less efficient, usually around 13%-16%. Why? Those multiple crystals can create boundaries, making it harder for electrons to move. But, on the plus side, they’re generally cheaper than monocrystalline cells.
- Thin-Film Cells: Here’s where it gets interesting. Thin-film cells have the lowest efficiency, around 7%-12%. But there’s a trade-off. They’re lightweight, flexible, and often cheaper to produce. Plus, they can be used in places the other two can’t, like on curved roofs or as portable panels.
In a nutshell, efficiency matters, but it’s not the only game in town. You’ve got to weigh it against cost, where you want to put the panels, and how you’ll use them.
The Role of Inverters: Converting Solar Energy to Usable Power
Understanding the Importance of Converting DC to AC
Solar panels work simply: they absorb sunlight, turning it into electricity. This electricity is called Direct Current (DC). Now, here’s the catch. Most gadgets, appliances, and systems in our homes and businesses run on Alternating Current (AC). So, there’s a conversion needed.
Why does it matter? DC electricity, while straight-forward, isn’t ideal for our daily needs. It’s great when distances are short and loads are constant. But for household purposes? Not so much. AC, on the other hand, can travel long distances without much loss of energy. It’s more flexible, playing well with the different demands of our appliances. So, we need to switch the solar panel’s DC output to AC.
The inverter is our switch guy. It takes in DC, processes it, and gives out AC. It ensures that the solar energy harvested is compatible with our home needs. Without inverters, the energy from solar panels would be much less useful.
How Inverters Integrate with Solar Panels for Home or Commercial Use
Solar panels on your roof? Great! They’re catching sunlight and turning it into DC power. But before this power can be used, it needs a tweak. The inverter is the tool that gives this tweak.
Here’s how it fits into the picture: The DC electricity from the solar panels flows into the inverter. The inverter then converts this DC into AC. This AC electricity then feeds into your home’s electrical system, ready to be used.
In home setups, you’ll often find a single, central inverter. It’s the hub, doing the DC to AC conversion for the entire solar panel system. But there’s another setup too, especially for bigger places or places with uneven sunlight. Here, instead of one big inverter, there are many small ones, called microinverters. Each solar panel gets its own microinverter. The advantage? Each panel can work independently, making the most of the sunlight it gets.
To sum it up, inverters are the bridge between the solar panels and your home. They ensure that the power coming from the panels is just right for your home appliances. Without them, the solar setup wouldn’t be half as useful.
Challenges and Advancements in PV Technology
Current Limitations in Efficiency and Storage
Solar panels are cool, but they’re not perfect. First, there’s the efficiency issue. Today’s common solar panels convert about 15-20% of sunlight into electricity. It means a lot of sunlight doesn’t get used. Why? Part of it is how solar cells work. They can’t use all the light spectrum. Only some parts. So, some energy just passes through.
Then there’s storage. Sun doesn’t shine all the time. So, you need to store extra solar power for later. Batteries are the go-to solution. But they’ve got problems. First, they’re expensive. Second, they don’t store a lot for a long time. Over time, batteries lose charge. And if there’s no sun for days? You might run out of power.
Recent Breakthroughs and What the Future Holds for Solar Technology
There’s good news on the horizon. Scientists are hard at work fixing solar power’s weak spots. On the efficiency front, new materials are coming out. Like perovskite. It’s a crystal. Put it with traditional solar cells, and boom! Efficiency jumps up. Some studies even say we might hit 40% efficiency soon.
For storage, things are changing too. The old lead-acid batteries? They’re giving way to lithium-ion ones. These new batteries are lighter, last longer, and can store more. There’s also research on flow batteries. They use liquid energy sources. They promise even longer life and more storage.
The future? It looks sunny for solar power. We’re seeing better panels and smarter storage. And as tech gets better, prices drop. Solar power is getting ready to be the big player in the energy game.
Conclusion
Back in the day, solar was expensive. Not many could afford it. Now? Prices are dropping. Fast. And as tech gets better, costs go down more. It’s simple math. Better tech + lower prices = more solar panels everywhere.
When we talk about living off the grid, solar energy really stands out, doesn’t it? It’s like having your own powerhouse, right on your roof or in your yard. You catch the sunlight, turn it into electricity. Sure, it’s a bit pricey to start, but think long-term; it pays off big time. So, to all the off-grid champs out there, solar energy is definitely a winner.